Characterisation
Risk assessment of known and new chemical compounds and materials is important to guarantee safety and sustainability during the development of new products. A key element of risk assessment of such compounds is their physical-chemical and biological characterization. With our characterisation solution package we provide new approach methods for characterisation of compounds, advanced materials and nanomaterials in the fields of toxicology and risk assessment.
- About
- About
- Physical characterisation of chemicals and materials
- Our solutions for you
- Our services
- Regulatory framework
- Request further information
- Order links
About
The foundation of modern risk assessment approaches is the characterisation of compounds and materials by which they are evaluated against specific key events for Adverse Outcome Pathways (AOPs). Thereby, the assessment process is structured in a four-step process including1:
- Hazard Identification (HI). During this step the molecular biology mechanisms are revealed which underlie adverse effects resulting from exposure to a test compound.
- Dose Response (DR). During this step the relationship between the dose of the test compound and its effect is determined using toxciokinetic and toxicodynamic models .
- Exposure Assessment (EA) which evaluates the possible exposure likelihood of organisms to the chemical including geographic place of uptake, biologic route of uptake (oral, per cutaneous uptake etc.) as well as the uptake-frequency describing how often an organism is exposed to the test chemical.
- Risk characterization which integrates all information from above to determine the overall risk of a chemical to an organism.
As we can draw from the four-step process for risk assessment above, one key element during the assessment process is in vivo studies in which the effect of a test compound on the organism is evaluated. In vivo studies are problematic in many ways. They are not only expensive to conduct and very time consuming, they also violate our scientific responsibility as scientists to protect animal welfare from an ethical and moral point of view. Therefore, moving away from animal experimentation is a great aim but very challenging since complex biological functions such as behaviour (a cause of complex neuronal cell activity) are not simple to mimic ex vivo. It is not only next generation in vitro approaches such as the multi organoid technology which seek solutions to study complex biological mechanisms ex vivo but also in silico methods are aiming to provide solutions for such an approach.2 In silico based risk assessment allows to take already existing data of known pharmacophores and their effect on cellular mechanisms and to apply this knowledge in Quantitative Structure-Activity Relationship (QSAR) models to characterise new compounds based on their chemical features including functional groups and molecular structure.3
Key for in silico based risk assessment is a robust data management infrastructure where workflows are standardised and harmonised by Defined Approaches (DAs) and by Integrated Approaches to Testing and Assessment (IATA); this approach ensures that evaluation and validation of compound resultss are conducted with high integrity to be able to trust resulting outcomes. An infrastructure for risk assessment is defined by the following elements:
- Access to Knowledge. Thereby knowledge is divided into two parts: The first part includes knowledge which is needed to conduct the assessment process and consists of Standard Operating Procedures (SOPs), regulatory guidelines, protocols, scientific publications etc. under which the characterisation of compounds and materials has to be examined. This knowledge can be organised in an easily accessible repository where all the documents are stored.The second part of knowledge includes all the information which is needed for the characterization process itself. This might include existing in vivo and in vitro data for the test chemical which has to be characterised. It also includes the knowledge about the test compound itself such as compound identity (CAS number, SMILES structure etc.), chemical information (functional groups, 3D structures, etc.) and information of biological activity (known pharmacology, known binding sites, etc.). This data is the data which will be used by the machine learning and artificial intelligence algorithms and which delivers the information based on which new compounds are characterised.
- Harmonised and standardised workflow. A harmonized and standardised workflow ensures the uniformity of the characterisation process and includes the creation of harmonised data throughout different approach methods. One example is the Recommended Metadata for Biological Images (REMBI) which is an international initiative to harmonise microscopy image data which comes from different microscopes so that it is easily Findable, Accessible, Interoperable and Reusable (FAIR). To make data FAIR, standardised templates for experimental protocols and correlating metadata should be elaborated and used.
- Data storage. Raw and processed data which has been generated throughout the experiments has to be stored in a safe and FAIR data repository. Especially for characterisation purposes, this data should be accessible with computational tools which allow the processing and analysing of all sorts of data formats including harmonised and customised metadata.
- Characterization output. The outcome of an evaluation can be structured in reports, charts, graphs, papers, heatmaps etc. Also this data has to be stored in a FAIR way and can be added to the existing repository as part of the knowledge base for that experiment.
One possible workflow for compound characterization is visualised in Figure 1 where a compound or material is synthesised and evaluated for specific endpoints in in vitro assays which are then analysed by computational algorithms to give a characterisation profile of that compound as an output.
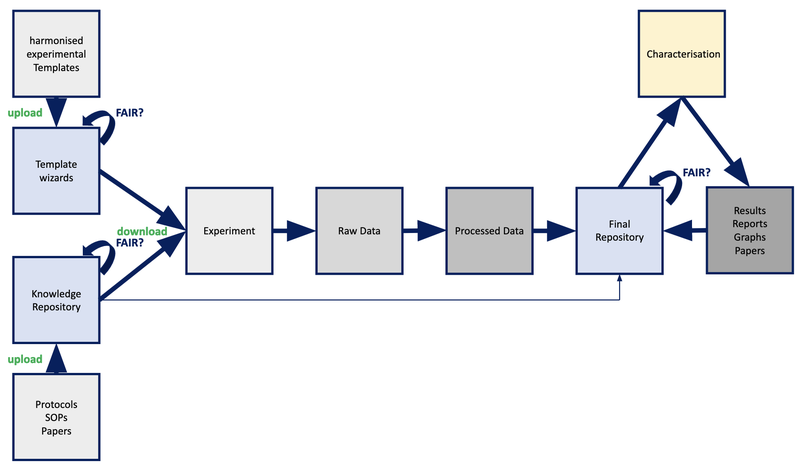
Figure 1: Possible work flow for a characterization process as described above.
Depending on the kind of repositories, templates, experiments and computational approaches, this characterisation infrastructure can be adapted and customised to specific needs for specific projects.
At SaferWorldbyDesign we have developed a characterisation infrastructure where we integrate newest data management approaches and computational technologies to guarantee efficient risk assessment due to your specific needs. If you want to learn more about our services, please see the «Our solutions» section on this page.
References
(1) A New Paradigm for Environmental Chemistry and Toxicology: From Concepts to Insights; Jiang, G., Liu, X., Eds.; Springer: Singapore, 2020.
(2) Cronin, M. T. D.; Madden, J. C.; Yang, C.; Worth, A. P. Unlocking the Potential of in Silico Chemical Safety Assessment – A Report on a Cross-Sector Symposium on Current Opportunities and Future Challenges. Computational Toxicology 2019, 10, 38–43. https://doi.org/10.1016/j.comtox.2018.12.006.
(3) Astuto, M. C.; Di Nicola, M. R.; Tarazona, J. V.; Rortais, A.; Devos, Y.; Liem, A. K. D.; Kass, G. E. N.; Bastaki, M.; Schoonjans, R.; Maggiore, A.; Charles, S.; Ratier, A.; Lopes, C.; Gestin, O.; Robinson, T.; Williams, A.; Kramer, N.; Carnesecchi, E.; Dorne, J.-L. C. M. In Silico Methods for Environmental Risk Assessment: Principles, Tiered Approaches, Applications, and Future Perspectives. In In Silico Methods for Predicting Drug Toxicity; Benfenati, E., Ed.; Methods in Molecular Biology; Springer US: New York, NY, 2022; Vol. 2425, pp 589–636. https://doi.org/10.1007/978-1-0716-1960-5_23.
Physical characterisation of chemicals and materials
One key element during risk assessment of chemicals and materials is to correlate biological effects, which are induced during the exposure of a test compound to a biological sample, with the physical properties of that compound. By this approach, compound - specific physical and chemical features can be linked to toxicity endpoints based on which new compounds can be identified as possible toxins.
Especially for nanomaterials, where the biological impact during exposure still remains unclear in most cases and where also regulations are still vague, one aim of modern risk assessment is to find new approach methods to be able to characterise nanoforms in concern of their potential toxic effect on organisms to guarantee consumer safety and sustainability of nanomaterial based products.
«Nanos» is the Greek word which means «dwarf» in English and what is the reason for why it is used to describe small structures. The präfix «Nano» describes the one billionth part of a physical unit for instance for force (nano-newton = 1.00*10-9 N), time (1 nano-second = 1.00*10-9 s) or space (1.00 nano-meter = 1.00*10-9 m). Thereby, a nanomaterial is defined such that it has at least one spatial dimension which is in the range of 1 to 100 nm in size. Nanoparticles like the C60 fullerene are thereby considered as zero-dimensional nanoparticles, since zero of their spatial dimension exceeds 100 nm. Carbon Nanotubes and nanohorns are considered as one dimensional nanomaterials since one of their spatial dimensions exceeds 100 nm. Based on the same principles there are two dimensional nanoforms, such as nanosheets and nanolayers as well as three dimensionally arranged nanoforms such as nanowire arrays and nanotube arrays. An overview over the different sorts of nanomaterials is given in Figure 1.
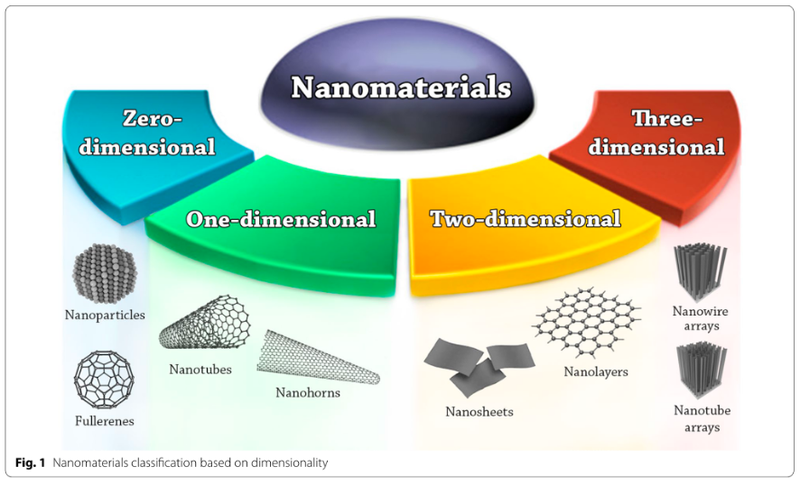
Figure 1: An overview over the different types of nanomaterials based on dimensionality.1
Since nanomaterials are in the size range of many biological gaps and pores, they can easily penetrate through biological barriers like the skin2 and even the blood brain barrier3 . Thereby, they reach regions where they can cause toxic effects leading to the outbreak of severe diseases such as neurodegenerative diseases.4 Nanotechnology is a relatively new field where new products are developed every day. The development of tools with which nanomaterials can be characterized in concern of safety reasons and their impact on the environment therefore is essential to ensure consumer safety and to protect the environment from toxic adverse effects by the increased use of those materials.
There exist a wide range of different nanomaterials. Many of them are found in nature as natural products and have been used by humans for thousands of years.1 One famous example is the Roman Lycurgus cup which contains gold and silver nanoparticles. It is green when illuminating it from the outside and red when illuminating it from the inside of the cup.1
Since nanoparticles are sized in the nanometer range, quantum effects appear where size dependent phenomena affect properties such as thermal properties, magnetic properties, electronic/optical properties and catalytic properties. This size dependent alteration of material based characteristics allows the development of new materials with new properties. Therefore, research as well as industry is currently driving forward the development of new non-natural nano-based products. One important application of such nanomaterials are nanomedicines for instance where nano based products serve as nano-drug-delivery-systems or where nanomaterials are used as treatment against cancer. Other applications include the usage of nanoparticles for catalysts, water treatment, energy storage, agriculture and much more.1
To assess the potential harm of nano-based materials on biological mechanisms and to elaborate regulations for the handling of nanoparticles based on the results which have been obtained during risk assessment, nanoparticles can be characterised based on their unique physical properties. Thereby, the following categories have been defined for which nanomaterials can be assessed for:
- Morphological and topographical characterisation
Endpoints: Size, Shape, Dispersity, Localization, Agglomeration, Surface morphology, Surface area, Pore size.
- Structural and chemical characterisation
Endpoints: Composition, Phase, Crystallinity, Functionalization, Oxidation, Surface charge, polarity, Bonding, Electrochemistry
- Mechanical characterisation
Endpoint: Toughness, Tensile strength, Compressive strength, Elasticity, Viscoelasticity, Hardness, Stiffness
- Optical and electrical characterisation
Endpoint: Absorbance, Reflectance, Fluorescence, Luminescence, Bandgap, electronic state, Photocativity, Electrical conductance
- Magnetical characterisation
Saturation magnetization, Remnant magnetization, Coercivity, g-Factor, Magnetic field intensity, Magnetic force, Magnetic susceptibility
- Thermal characterisation
Endpoint: Melting point, Crystallization point, Structural phase transition, Heat capacity, Thermal conductivity, Thermal stability, Oxidative stability
There are alot of different tools which allow the characterization of nanomaterials for the mentioned endpoints. Table 1 gives an overview over which techniques are used for which type of characterization and for which endpoint.
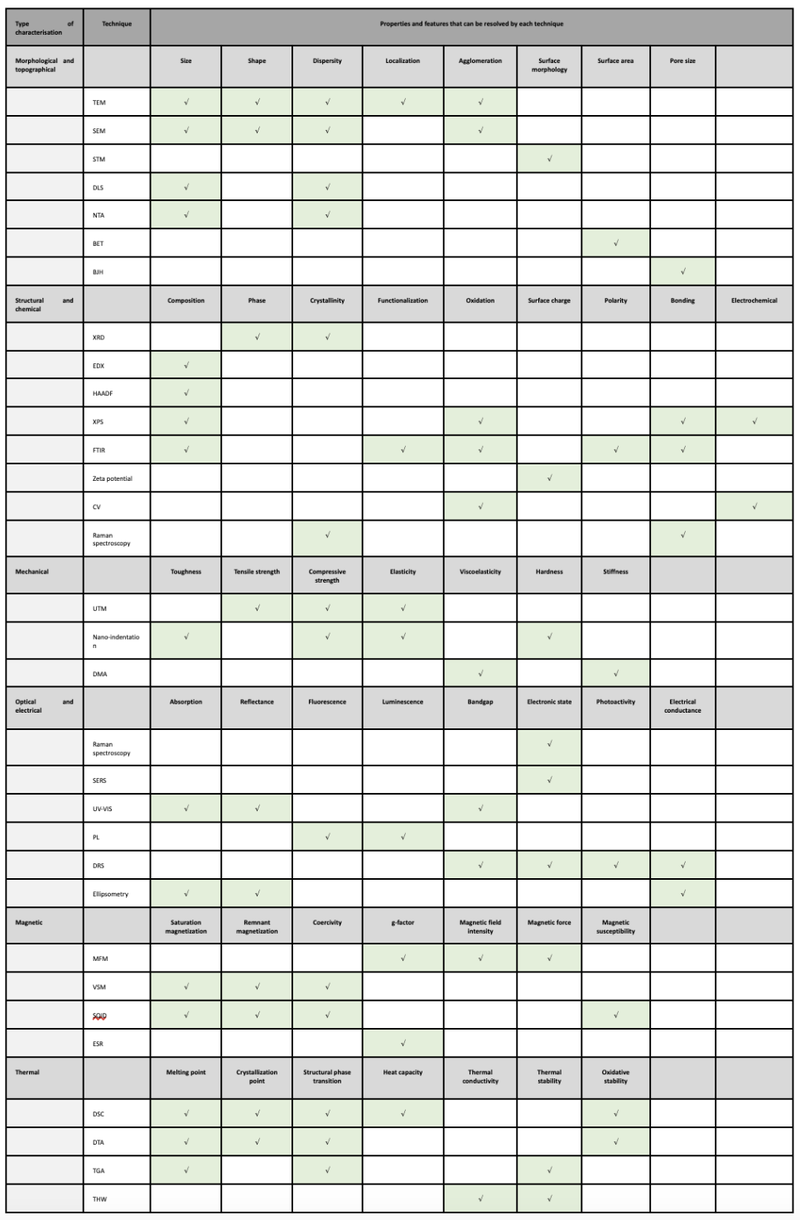
Table 1: Overview over the characterisation methods for the different types of characterisation and their endpoints.1 TEM = Transmission Electron Microscopy, SEM = Scanning Electron Microscopy, STM = Scanning Tunneling Microscopy, DLS = Dynamic Light Scattering, NTA = Nanoparticle Tracking Analysis, BET = Brunauer-Emmett-Teller method, BJH = Barrett-Joyner-Halenda method, XRD = X-Ray diffraction, EDX = Energy-dispersive X-ray spectroscopy, HAADF = High-angle annular dark-field imaging, XPS =X-Ray photoelectron spectroscopy, FTIR = Fourier-transform infrared spectroscopy, CV = Cyclic Voltammetry, UTM = Universal Testing Machine, DMA = Dynamic Mechanical Analysis, SERS = Surface-enhanced Raman spectroscopy, PL = Photo-luminescence, DRS = UV-VIS diffuse reflectance spectroscopy, MFM = Magnetic Force microscopy, VSM = Vibrating-sample magnetometry, SQUID = Superconducting Quantum Interference Device magnetometry, ESR = Electron Spin Resonance Spectroscopy, DSC = Differential Scanning Calorimetry, DTA = Differential thermal Analysis, TGA = Thermogravimetric Analysis, THA = Transient Hot Wire method.
One example of nanomaterial based risk assessment is the recently started European ACOORDs project5 where also SaferWorldbyDesign takes a leading role. The aim of the project is to synthesise a variety of functionalized graphenes (2D nanomaterials). The nanomaterials will be characterised by assays in concern of their biological impact and then imaged by different imaging techniques. With this, tools can be developed which categorise 2D nanomaterials based on features which are extracted from images and hence offer an effective, straight forward and fast characterisation procedure for next generation risk assessment.
References
- Characterization, and Applications: A Comprehensive Review for Biologists. J Nanobiotechnol 2022, 20 (1), 262. https://doi.org/10.1186/s12951-022-01477-8.
- Meng, H.; Leong, W.; Leong, K. W.; Chen, C.; Zhao, Y. Walking the Line: The Fate of Nanomaterials at Biological Barriers. Biomaterials 2018, 174, 41–53. https://doi.org/10.1016/j.biomaterials.2018.04.056.
- Sharma, H. S.; Hussain, S.; Schlager, J.; Ali, S. F.; Sharma, A. Influence of Nanoparticles on Blood–Brain Barrier Permeability and Brain Edema Formation in Rats. In Brain Edema XIV; Czernicki, Z., Baethmann, A., Ito, U., Katayama, Y., Kuroiwa, T., Mendelow, D., Eds.; Acta Neurochirurgica Supplementum; Springer Vienna: Vienna, 2010; Vol. 106, pp 359–364. https://doi.org/10.1007/978-3-211-98811-4_65.
- Mushtaq, G.; Khan, J.; Joseph, E.; Kamal, M. Nanoparticles, Neurotoxicity and Neurodegenerative Diseases. CDM 2015, 16 (8), 676–684. https://doi.org/10.2174/1389200216666150812122302.
- Publisher. News - Nano: European project investigates sustainability of the material of the future graphene. https://www.bam.de/Content/EN/Press-Releases/2023/Materials/2023-05-08-nano-project-sustainability-graphene.html (accessed 2023-06-07).
Our services
At SaferWorldbyDesign we developed an integrated characterisation infrastructure. Implemented in this infrastructure are the following tools:
The eNanoMapper environment, which includes a database containing protocols and experimental templates which are standardised and harmonised to make data generated throughout the experimental process easily Findable, Accessible, Interoperable and Reusable (FAIR) what is key to ensure integrity and validity of experimental outcomes. The eNanoMapper environment also provides a FAIR user guide which helps you to make your data FAIR.
The ACENano Infrastructure is a knowledge base which manages protocols, SOPs, articles and linked datasets which can be explored through a user-friendly interface. It allows you to collect and organise knowledge which is needed to conduct characterisation experiments.
EdelweissData is a data management infrastructure solution developed and provided by Edelweiss Connect. It can provide a repository where your data can be stored in a FAIR and safe way with high integrity and harmonisation. Specific to your needs we can develop computational tools such as observable notebooks and user friendly interfaces to easily manage your data and its analysis.
Computational characterisation tools which are based on the newest machine learning and artificial intelligence technologies. Thereby, we analyse your raw or processed data for your endpoint of interest and summarise the outcoming results for instance in detailed reports, graphs and heatmaps. We also integrate knowledge in knowledge graphs where the newly generated knowledge can be explored interactively.
With this we offer a characterisation workflow that covers all needs from the beginning of the assessment process throughout the end. One possible workflow is visualised in Figure 1, showing the procedure from template development and knowledge management over the experimental phase throughout the analysis of the experimental results.
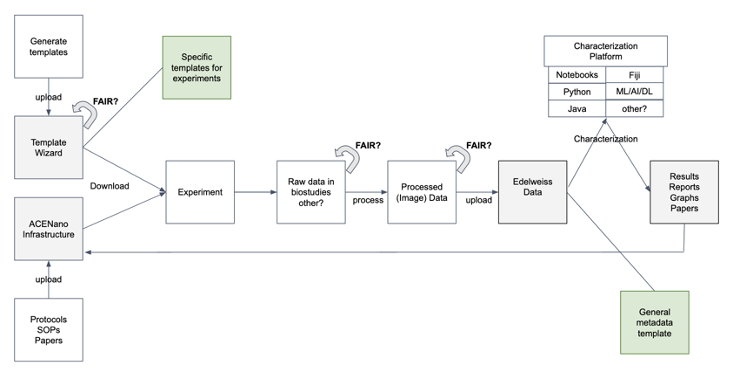
Figure 1: Possible work flow for a characterization process at SaferWorldbyDesign as described above.
Furthermore, we provide a large variety of next generation in vitro and in silico technologies in collaboration with our partners. Those technologies include organ specific cell and tissue models such as the HepaRG cell model provided by Biopredic to conduct liver specific experiments or the multi organ on a chip model provided by TissUse with which compounds can be characterised in a multi organ in vitro environment. Also computational characterisation toolboxes such as the MultiCASE CASE Ultra toolbox developed by MultiCASE are implemented in our solutions. MultiCASE CASE Ultra is a toolbox with which compounds can be characterised in a QSAR approach against different endpoints such as skin irritation, skin sensitisation, endocrine disruption and much more. To explore the whole collection of services which we offer, please visit the «partners» section on this homepage.
Here you come to the partners section: Link
To provide a good and user-friendly overview over our solutions, we collect our services in solution packages for specific organ endpoints such as skin, liver, kidney etc. which you can explore in the «solutions» part of this portal. Thereby, we can adapt our approaches and services to your specific needs and interests to always provide you with the best solution that serves you the best. If you are interested in taking advantage of our services, please feel free to reach out to us.
Regulatory framework
Defined Approaches (DA) and Integrated Approaches to Testing and Assessment (IATA) which are defined and controlled by authorities are key to ensure comparable and valuable characterisation results during the risk assessment process for test compounds to ensure their integrity, trust and validity. Therefore, the assessment processes at SaferWorldbyDesign are based on such guidelines. One example is SaferSkin where the OECD has elaborated detailed procedures1 which have to be followed to characterise compounds in concern of their potential to induce skin sensitisation and which we have implemented in our assessment process. For characterising compounds as part of the risk assessment process, the following guidelines are important:
- The OECD Guidelines for the Testing of Chemicals
- The OECD series on testing and assessment
- United States Environmental Protection Agency (EPA) Science Policy Council Handbook on Risk Characterization
References
(1) OECD. Guideline No. 497: Defined Approaches on Skin Sensitisation; OECD Guidelines for the Testing of Chemicals, Section 4; OECD, 2021. https://doi.org/10.1787/b92879a4-en.